Difference between revisions of "Starburst Amacrine Cell"
Line 1: | Line 1: | ||
+ | <translate> | ||
+ | |||
[[File:Keeley SACs.jpg|thumb|right|350px|Images of starburst amacrine cells showing the "starburst" shape of the dendritic arbor<ref>Keeley, P.W. et al. Dendritic spread and functional coverage of starburst amacrine cells. J. Comp. Neurol. 505, 539–546 (2007).</ref>.]] | [[File:Keeley SACs.jpg|thumb|right|350px|Images of starburst amacrine cells showing the "starburst" shape of the dendritic arbor<ref>Keeley, P.W. et al. Dendritic spread and functional coverage of starburst amacrine cells. J. Comp. Neurol. 505, 539–546 (2007).</ref>.]] | ||
Line 160: | Line 162: | ||
{{Retinal Neuron Types}} | {{Retinal Neuron Types}} | ||
+ | |||
+ | </translate> |
Revision as of 03:29, 24 June 2016
Starburst amacrine cells (SAC or SBAC) are, as the name would suggest, a subtype of retinal amacrine cells primarily identified by the characteristic “starburst” shape of the dendritic arbor. Two main roles for starburst amacrine cell have been characterized. SACs are (1) important in the computation of direction-selectivity and they also serve (2) an important function in the development of the retina. There are two subtypes of starburst amacrine cells, unambiguously defined by their differential stratification, morphology, connections, and roles in direction-selectivity.
Contents
Physiology
Visual response properties
Generally speaking, starburst amacrine cells (SACs) respond to a visual stimulus that moves from the soma (cell body) towards the distal dendrites (centrifugal or CF motion) but not to a visual stimulus moving in the opposite direction (centripetal or CP motion). This property of SACs was established by two photon imaging.
The figure below shows a diagram of the visual response properties of a starburst amacrine cell. (A) Response to a flash of light on the distal dendrites. (B) Response to a proximal flash of light followed by a distal flash of light, simulating centrifugal motion. (C) Response to a distal flash of light followed by a more distal flash of light, simulating centripetal motion. Note that the SAC gives the greatest response to motion in the centrifugal direction. [2]
Cellular biophysics
Starburst amacrine cells are the only cells in the retina that have been shown to release two neurotransmitters. They secrete the normally inhibitory neurotransmitter GABA and the excitatory neurotransmitter ACh. Release of both neurotransmitters is monosynaptic and mediated by calcium. However, it has also been shown that these two neurotransmitters are not released together. The release properties of the two transmitters are affected differently by alterations in the buffer, specific calcium blockers, and extracellular concentrations.
Connection Asymmetry
Direction selective ganglion cells (DSGCs) receive inputs from many starburst amacrine cells, but the type or strength of these connections are importantly asymmetric. DSGCs have a preferred direction and a null direction, meaning that they will respond only to a visual stimuli that moves in the preferred direction.
- GABA: If you stimulate (depolarize) a starburst amacrine cell that first connects with a DSGC along its null direction, you inhibit the DSGC in a GABA-mediated way. If you depolarize a starburst amacrine cell that first connects with a DSGC along the preferred direction, you don't get inhibition. Thus, DSGCs recieve asymmetric GABAergic (GABA-mediated) inputs from SACs.[3]
- ACh: If you stimulate (depolarize) a starburst amacrine cell that connects to a DSGC in any direction you will excite the DSGC in an ACh-dependent way. Thus, DSGCs receive symmetric cholinergic (ACh-mediated) synapses from SACs.[3]
The asymmetric GABA connections are essential for the computation of direction selectivity. If you block the GABA channels in the DSGC, you ablate direction selectivity. However, if you just block the cholinergic channels in the DSGC you don't ablate direction selectivity but merely reduce all responses by about half. Thus, the exact cellular function of ACh is still unknown.
Anatomy
Starburst amacrine cells have a very specific anatomy. Their descriptive name arose from the earliest images of this cell type, in which the characteristic 'starburst' branching of the dendritic arbor could be seen. There are two subytpes of amacrine cells, types a and b.[4] In many categories of cells, classification of subtypes can be inexact and somewhat subjective. For SACs, however, the two subtypes display marked difference in location, shape, and connections, with these structural differences playing a major role in their function. In fact, it was the differential stratification between subytpes that helped identify starburst amacrine cells as the acetylcholine secreting cells in the retina.[5]
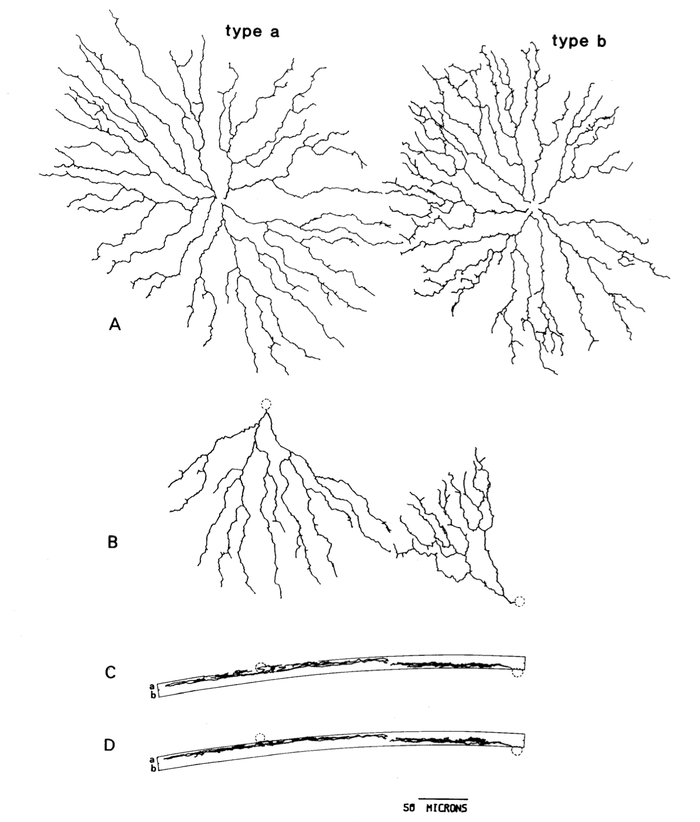
Location
Stratification
The distinct stratification of starburst amacrine cell subtypes was fundamental to the identification of starburst amacrine cells (SAC) as the source of acetylcholine in the retina. By the late 1970s, it was known that acetylcholine (ACh) was synthesized from choline in two different populations of cells in the retina. These ACh synthesizing cells were putatively identified as amacrine cells, since about half of them had cell bodies where one would normally expect to find amacrine cells, flanking the inner plexiform layer (IPL).[6] These were called type a (OFF) starburst amacrine cells, since they were observed to branch in IPL sublamina a. The other half had their cell bodies in the ganglion cell layer (GCL) but did not appear to be ganglion cells. Since their cell bodies were "displaced" to the ganglion cell layer and they were observed to branch in IPL sublamina b, they are referred to as both type b (ON) or displaced starburst amacrine cells.[5] It had previously been shown that starburst amacrine cells also displayed the same localization pattern, and further study confirmed that the starburst amacrine cells were indeed the cholinergic cells in the retina.

Tiling
Another important aspect of SAC localization is that there is a high degree of overlap (often called tiling overlap) between the arbors of neighboring starburst amacrine cells.[7] The dendrites of many cell types will detect, during development, when they interact with dendrites of another cell and will stop growing. This results in a precise segregation of dendritic arbors that do not overlap. When this non-overlapping system happens, you end up with a tiling factor of one, meaning that the the total area covered by all the cells combined equals the total area. Many amacrine subtypes have a tiling factor of one. Starburst amacrine cells, however, can have a tiling factor of around 100, meaning that the overlap between dendritic arbors is so high that the sum of the area occuped by all cells is 100 times greater than the total area. A high tiling factor can also be referred to as high eccentricity. In the figure to the right, each color represents a preferred direction and solid circles represent the soma of individual SACs. Note the degree of overlap between neighboring cells.
Shape
Starburst amacrine cells are named for the highly distinctive shape of their dendritic arbor. The average cell body diameter of a SAC is approximately 9 to 12 micrometers, while the dendritic field size ranges from about 150 micrometers (in the visual streak; see shape subsection below) to 600 micrometers (in the dorsal retina).[6] The dendritic arbor is sufficiently regular to allow for the precise characterization, which was formalized in the early 1980's.[6] The branching pattern originates from 4 or 5 "primary dendrites" extending from the cell body. Said branching is "concentrically and evenly distributed about the cell body" and there is almost no overlap of dendrites. The dendritic tree has been divided into three "distinct annular zones" as ones progresses from the cell body radially outwards.
Regions of the Dendritic Arbor
- Proximal Region (Proximal)
The first zone, closest to the cell body, is known as the proximal region. The dendrites here are relatively thin, with a diameter not greater than 1.5 micrometers at their origin. These dendrites taper as we progress radially outwards, until the region ends at the third or fourth branch point. The diameter of these dendrites is distinctly smaller in the visual streak (see shape subsection below), as opposed to gradually decreasing is size as we move away from the streak- as is the case for dendrites of the other two regions.[6]
- Intermediate Annular Region (Intermediate)
The dendrites will suddenly become more slender around the third or fourth branch point, which is where this region begins. This region is characterized by uniform dendrites.[6]
- Distal Annular Zone (Distal)
This zone is characterized by slender but uniform segments about 0.2 micrometers in diameter that are interrupted by "aperiodic preterminal and terminal varicosities and boutons," which range from 1 to 1.5 micrometers in diameter.[6] The distal zone has recently been shown to be the region of the dendritic arbor where direction selectivity takes place[8]. See the physiology section for more information.
Subtype Differences
There are some distinct structural differences between subtypes a and b, several of which can be seen from the figure at the beginning of this section. Generally speaking, the type a cell has a larger dendritic arbor and has a more regular branching pattern.[5] Type a has fewer "branches, spines and boutons in the distal dendritic zone"[5].

Relationship to Visual Streak
The shape of SACs is also highly correlated to their location in the retina with respect to the Visual Streak (or fovea, depending on the species).[5] In many species, the area of greatest acuity in the retina is not a single point (fovea), but rather an elongated "streak" running across the retina. In all species studied, the area of highest acuity (whether fovea or visual streak) boasts the highest concentration of cones, the lowest concentration of rods, and much smaller receptive field sizes for all cells. It is unsurprising that starburst amacrine cells also display structural changes as the perpendicular distance from the visual streak increases. Nearest the visual streak, both type a and b SACs have a relatively small cell body size and dendritic field diameter as well as a relatively high frequency of branching and frequency of synaptic boutons.[5] As we move perpendicularly away from the visual streak, cell body size and dendritic field diameter increases, while the frequency of branching and synaptic boutons decreases. (See figure.)
Connections
At its basic level, the “vertical” flow of information in the retina goes from photoreceptors (input) to bipolar cells to ganglion cells (output). Amacrine cells in general affect this circuit “horizontally” at the level of the bipolar to ganglion cell connection, typically receiving input from bipolar cells and making synapses on both bipolar cells and ganglion cells.
A typical neuron consists of a cell body, dendrites (dendritic arbor), and an axon (axonal arbor). Neurons will typically receive inputs on their dendrites (postsynaptic) and will output information on their axon (presynaptic). Many types of amacrine cells- including starburst amacrine cells- do not follow this structural or functional pattern. Many of these cells make both input and output connections along the same neuronal processes that show no distinction between axon and dendrite, and are nevertheless called "dendrites." Starburst amacrines are one of the types that do not have axons and both receive and transmit information via their dendrites.[8] In the figure below, you can see a comparison between three types of amacrine cells. [9]
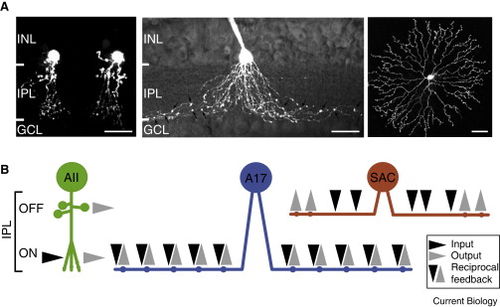
Basic Circuitry
At the most basic level, starburst amacrine cells will receive inputs from bipolar cells and will output information to ganglion cells. There are also connections (inhibitory) between starburst amacrine cells, whose dendritic arbors have substantial overlap. The circuit is mirrored in ON and OFF (types b and a) starburst amacrine cells. Here's how it works in an ON starburst amacrine cell. ON bipolar cells make excitatory synapses to an ON starburst amacrine cells. In the distal portions of the dendrites, the ON starburst amacrine cell makes inhibitory synapses to a direction-selective ganglion cell. For a more complete description of the function of this circuit, see the physiology section.
Direction Selectivity
It has been known for almost five decades that there are ganglion cells in the retina that respond preferentially to a stimulus moving in a particular direction. These ganglion cells, termed direction-selective ganglion cells have been extensively studied and characterized. However, it was not known for for many years whether the computation of direction selectivity occurred postsynaptically (i.e. in the ganglion cells themselves) or in some presynaptic partner of the ganglion cells. Starburst amacrine cells, which had been shown to be presynaptic to the DSGCs, were an ideal candidate for a presynaptic calculator of direction selectivity. Ultimately, it was shown that direction selectivity does occur in the distal dendrites of starburst amacrine cells.
Above we can see a diagram of the circuit elements involved in direction selectivity. Note that the ON and OFF subtypes of SACs have their cell bodies flanking opposite sides of the inner plexiform layer (IPL). The ganglion cells all have their cell bodies below the IPL in the ganglion cell layer (GCL), but where they send their projections is determined by whether their direction selectivity is ON or OFF. An ON direction selective cell will send its projections to sublamina b (the lower sublamina in this figure). Likewise, an ON starburst amacrine cell will send its projections to sublamina b[2]
Wiring Symmetry and Asymmetry
Starburst amacrine cells have long been known to be the presynaptic partners to direction selective ganglion cells, and in fact have been shown to be the cells that actually compute the direction selectivity.[3] In particular, a starburst amacrine cell will make synapses with many DSGCs, but the region of the SAC dendritic arbor that will synapse with a particular DSGC is dependent on the direction of selectivity of that particular DSGC. For example, the "rightmost" part of the dendritic arbor of a SAC will preferentially synapse with DSGCs having a "leftward" direction selectivity.[10] (see figure below)
Let's consider the rightmost part of a SAC dendritic arbor. It will preferentially make synapses with a left-selective ganglion cell. This computes direction selectivity because when a stimulus moves in a rightward direction, the SAC is being centrifugally stimulated and will provide maximum inhibition. When light is moving in a leftward direction, the SAC is being centripetally stimulated and will provide minimum inhibition.
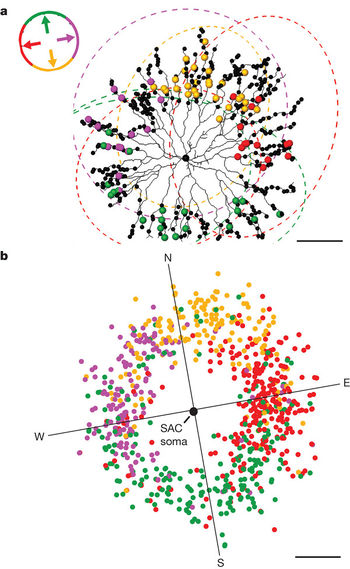
Current Model
So how is direction selectivity computed? The image below diagrams the current prevailing model.[2] As discussed above, SACs receive inputs on their proximal dendrites- both excitatory synapses from bipolar cells and (GABAergic) inhibitory synapses from other starburst amacrine cells. They also make (GABAergic) inhibitory synapses on their distal dendrites both to other starburst amacrine cells and to direction selective ganglion cells. SACs will preferentially connect with DSGCs as described in the wiring symmetry subsection (not shown in this figure). Furthermore, a SAC will provide greater inhibition when visually stimulated by centrifugal motion compared to centripetal motion.
Development
The retinal circuitry of most mature organisms is notably different from the circuitry established during development. This early wiring is not simply an immature state of the developing system. Rather, specific connections and cellular properties are established during early development that are completely different from those properties seen in the mature system. In fact, this early circuitry must be "deconstructed" during postnatal development prior to completion of the final visual circuit. Starburst amacrine cells have unique properties during development and play an important (but poorly understood) role in the signals generated by this early system.
Spiking Properties
The canonical neuron transmits information through the generation of action potentials. In the retina, however, many cell types including SACs do not generate action potentials. Rather, graded depolarization is sufficient to transmit information and only the output cells of the retinal circuit create action potentials. During development, however, several of these normally non-spiking cell types do generate action potentials. Starburst amacrine cells transition late in development from a spiking to a non-spiking state, and this transition has been shown to occur though a loss of voltage-gated sodium channels.[11]
The figure above shows the generation of action potentials by starburst amacrine cells in the early retina. Note that as development increases (left) the spiking of the SACs is completely abolished.
Acetylcholine
In the mature retina, SACs are not themselves responsive to acetylcholine. Rather, synapses between SACs in the mature retina are GABA-dependent and only the specific targets of SACs (i.e. direction selective ganglion cells) contain acetylcholine receptors. In the early retina, however, many cells contain nicotinic acetylchole receptors (nAChR) and are therefore responsive to ACh. Even starburst amacrine cells themselves are responsive to ACh.[12]
Furthermore, the mechanism of transmission of ACh is dramatically different. In this early circuit, true synapses have yet to form. The transmission of ACh occurs in what is called a "volume" fashion, where a large amount of ACh is released by a cell and diffuses large distances without a specific "postsynaptic" target.[12] Since many cells in the early retina contain AChR, spontaneous activity of a SAC can therefore activate a large number of cells.
Retinal Waves
There are several interesting features of neuronal activity in the retina. First, it is spontaneously generated. This was first shown to occur in ganglion cells, and was then demonstrated to occur in starburst amacrine cells as well.[13] Spontaneously generated signals have been shown to be important in generating proper connectivity in various parts of the central nervous system. The second interesting fact is that, in the retina, these spontaneous signals do not propagate across the entire retina but rather are restricted to non-intersecting, dynamic "domains." A group of cells in the retina will periodically fire in a way that resembles a propagating "wave" across a portion of the retina. Over time, if you record from the entire retina, you can see that a "mosaic" pattern emerges where the retina is segregated into a variety of such domains. There also appears to be a period, perhaps of inhibition, following a given wave before another can be generated in the same region.
It has been shown that acetylcholine is important in, and possibly responsible for, the generation of retinal waves. This makes sense if we consider the volume method by which ACh is released in the early retina. ACh diffuses over a large area, and many different cells have ACh receptors. Thus, SACs are a strong candidate for initiation of a signal which covers such a large region.
Early spontaneous neural signaling is important in the establishment of final neural circuitry. The precise importance of acetylcholine (and by extension, SACs) to the development of the retina is not well understood. SACs have some influence on dendritic formation in ganglion cells, but abolishing the nicotinic acetylcholine receptors from the retina does not prevent the formation of a final circuit. See the 2012 review for a more detailed discussion.[12]
Mosaic of retinal waves: In the above figure, each color represents a particular "domain" of activation.[13] Note that, as time progresses, the domains remain spatially segregated but their locations shift.
Developmental Stages
It turns out that there is not one, but three separate developmental circuits that form in the retina. As discussed above, a cholinergic network forms in the immature retina that is important in the generation of retinal waves. However, there is a stage before this in which retinal waves are generated by gap junctions (or thought to be) and a stage following it in which retinal waves are facilitated by glutamatergic connections.[12]
Early in development, retinal waves are thought to be generated by gap junctions (left panel of A, above). Later in development, the cholinergic network forms and SACs generate retinal waves (center panel of A). Just before completion of the mature circuit, the cholinergic network is deconstructed and glutamateric connections become responsible for the retinal waves (right panel of A).[12] Part B of the figure shows changes that occur if you prevent each of the stages. The topmost line represents the wildtype transition between the three stages. If you prevent the cholinergic stage, the gap-junction stage lasts longer. If you prevent the glutamatergic stage, the cholinergic stage lasts longer.
History
Starburst amacrine cells were identified in the 1970s on the basis of the distinct "starburst" shape of their dendritic arbor. Later and completely independently, it was first discovered in 1976 that there were cells in the rabbit retina that secreted the neurotransmitter acetylcholine and were thus referred to as cholinergic neurons. The most likely candidates were bipolar cells and amacrine cells, but it was not known at the time that the cholinergic neurons of the retina and SACs were one in the same. Later in 1976, it was shown that ganglion cells were postsynaptic to the cholinergic neurons.[14] By the 1980s, there was strong evidence that these two populations of cells were one and the same, largely on the basis of the stratification of subtypes. (See the structure section.)
In the late 1990's, the role of starburst amacrine cells in development became an area of interest. It was discovered that they served a completely different function in the developing retina- generating retinal waves (see development section).
Perhaps the most studied area of starburst amacrine cell research is in relation to direction selectivity. The connectivity between direction selective ganglion cells and SACs was established early on. Although there was some early controversy regarding the role of SACs in the generation of direction selectivity, there is now consensus regarding their pivotal role.
Open Questions
As Richard Masland notes, there are "some interesting mysteries" regarding starburst amacrine cells.[15] SACs are especially noteworthy for secreting both acetylcholine and GABA. Over the years the function of GABA has been extensively studied in these cells, especially in relation to the connections between SAC and direction-selective ganglion cells. However, very little is known about the role of ACh, the second neurotransmitter. Although the release of GABA from SACs is known to be important in direction selectivity, "no one really knows why they [SAC] release acetyl choline- even though that’s how they were first discovered." [15]
Some other mysteries to ponder:
- What is the true significance of ACh in the development of the retina in general and in the propagation of retinal waves in particular? Why do so many cell types express AChR during development? Why does the retinal pass through three phases of circuitry?
- Why do the starburst amacrine cells express only one isoform of GAD? Why is it is the less common isoform?
- How to the SACs decide which ganglion cells to synapse on during development such that the connective asymmetry is created?
Relation to Eyewire
Many complete starburst amacrine cells have been reconstructed as part of EyeWire.
References
- ↑ Keeley, P.W. et al. Dendritic spread and functional coverage of starburst amacrine cells. J. Comp. Neurol. 505, 539–546 (2007).
- ↑ 2.0 2.1 2.2 2.3 Vaney, E.I., Sivyer, B., and W.R. Taylor (2012) Direction selectivity in the retina: symmetry and asymmetry in structure and function. Nature Reviews Neuroscience. 13: 194-208
- ↑ 3.0 3.1 3.2 Taylor, W. R. and R. G. Smith (2012) The role of starburst amacrine cells in visual signal processing. Visual Neuroscience. 29: 73-81.
- ↑ Famiglietti EV., Jr. ‘Starburst’ amacrine cells and cholinergic neurons: mirror-symmetric on and off amacrine cells of rabbit retina. Brain Res. 1983;261:138–144.
- ↑ 5.0 5.1 5.2 5.3 5.4 5.5 5.6 5.7 Famiglietti, E.V. (1985) Starburst amacrine cells: morphological constancy and systematic variation in the anisotropic field of rabbit retinal neurons. J. Neurosci. 5, 562–577
- ↑ 6.0 6.1 6.2 6.3 6.4 6.5 Famiglietti, E. V., Jr. (1983) ON and OFF pathways through amacrine cells in mammalian retina: The synaptic connections of “starburst” amacrine cells. Vision Res. 23: 1265-1279.
- ↑ Tauchi, M. & Masland, R. H. The shape and arrangement of the cholinergic neurons in the rabbit retina. Proc. R. Soc. Lond. B 223, 101–191 (1984).
- ↑ 8.0 8.1 8.2 Euler, T., Detwiler, P.B., and Denk, W. (2002). Directionally selective calcium signals in dendrites of starburst amacrine cells. Nature 418, 845–852.
- ↑ 9.0 9.1 Schubert, T., and Euler, T. (2012). Retinal Processing: Global Players Like it Local. Curr. Biol. 20, 486-488. Paywalled.
- ↑ 10.0 10.1 Briggman, K.L., Helmstaedter, M. & Denk, W. Wiring specificity in the direction-selectivity circuit of the retina. Nature 471, 183–188 (2011).
- ↑ Zhou, Z.J. & Fain, G.L. (1996). Starburst amacrine cells change from spiking to nonspiking neurons during retinal development. Proceedings of the National Academy of Sciences of the United States of America 93, 8057–8062.
- ↑ 12.0 12.1 12.2 12.3 12.4 Ford, K.J. and M. B. Assembly and disassembly of a retinal cholinergic network. Visual Neuroscience. 29, 61-71 (2012).
- ↑ 13.0 13.1 Feller, M.B., Wellis, D.P., Stellwagen, D., Werblin, F.S. & Shatz, C.J. (1996). Requirement for cholinergic synaptic transmission in the propagation of spontaneous retinal waves. Science 272, 1182–1187.
- ↑ Masland RH, Ames A,, 3rd. Responses to acetylcholine of ganglion cells in an isolated mammalian retina. J Neurophysiol. 1976;39:1220–1235.
- ↑ 15.0 15.1 Conversation with Richard Masland 4/6/12
|